In this paper, the effect of gas cooling on microstructure refinement of single crystal blades produced by DGCC gas cooling casting process was studied. The primary dendrite arm spacing (PDAS) reaches the highest value on the airfoil and the lowest value on the blade platform. However, when Bridgman’s method is used, the PDAS value changes along the blade in the opposite direction. The DGCC gas cooling casting method results in a reduction of about 100 μm in the PDAS value in the blade platform compared to conventional radiant cooling.
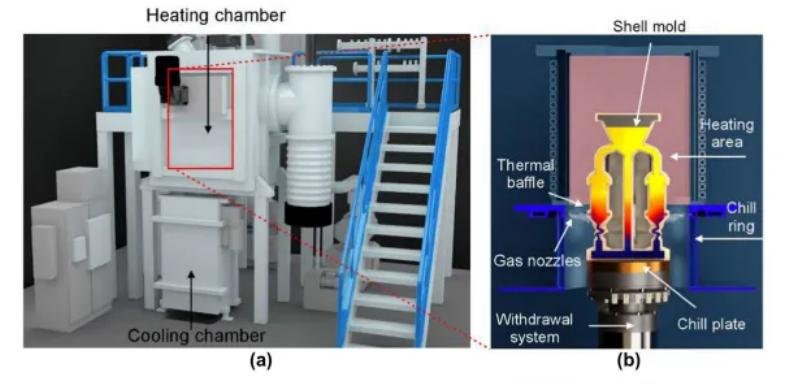
In the process of directional solidification of nickel-based superalloy, the dendrite structure is refined by decreasing the primary dendrite arm spacing (PDAS) and increasing the axial temperature gradient at the solidification front, so as to improve the operating temperature and mechanical properties of single crystal blades. In the Bridgman method, the radiant heat transfer between the workpiece and the furnace severely limits the effectiveness of mold shell cooling, thus reducing the temperature gradient and not conducive to dendrite microstructure refinement. Therefore, in order to improve the single crystal quality and process yield, alternative methods of directional solidification have been developed, such as liquid metal cooling (LMC) , gas cooling casting (GCC), downward directional solidification (DWDS) and fluidized carbon bed cooling method (FCBC).
In the above mentioned methods, in addition to radiation cooling, convection cooling is mainly used to improve the heat extraction efficiency of the mold shell surface. In liquid metal cooling (LMC) and fluidized carbon bed cooling (FCBC) methods, the mold shell is immersed in a cooling bath and a fluidized bed, respectively. In gas-cooled casting (GCC) and downward directional solidification (DWDS) methods, gas is injected into the shell surface to cool the casting as it moves from the furnace heating zone. The continued development of blade production methods using inert cooling gases shows the great potential of these methods, as the cost is relatively low compared to the LMC liquid metal cooling method, while the microstructure of the workpiece is improved compared to the Bridgman method. Konter et al. demonstrated a method for making large gas turbine (IGT) blades using inert cooled gases, while Wang et al. used this method to produce small aviation turbine blades. This is enough to prove that the use of inert cooling gas is an effective way to effectively improve the temperature gradient and refine the dendrite structure. Although these methods are effective, they may have very limited applications in blade manufacturing on an industrial scale, especially where multiple castings are placed simultaneously in complex die casings.
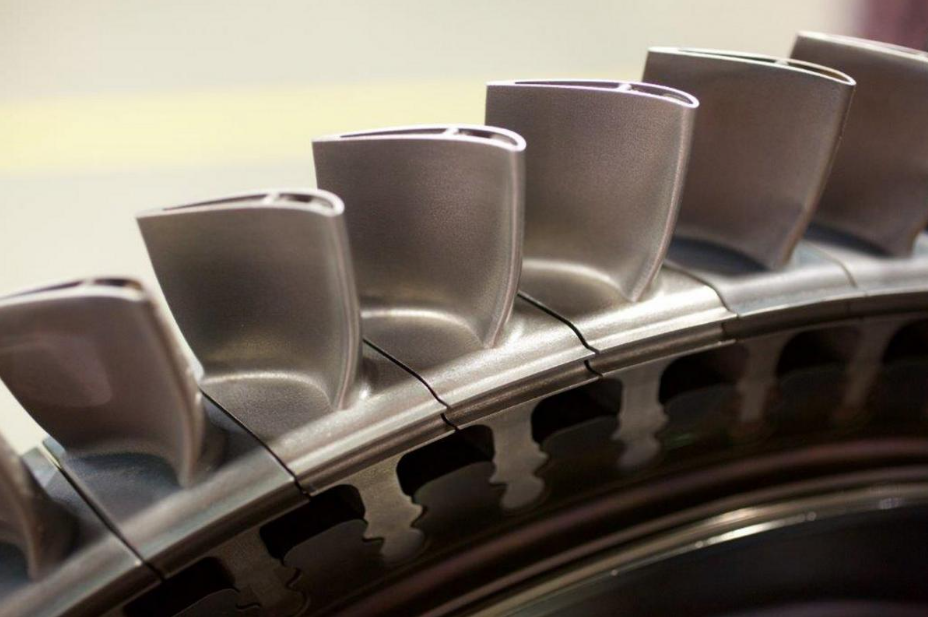
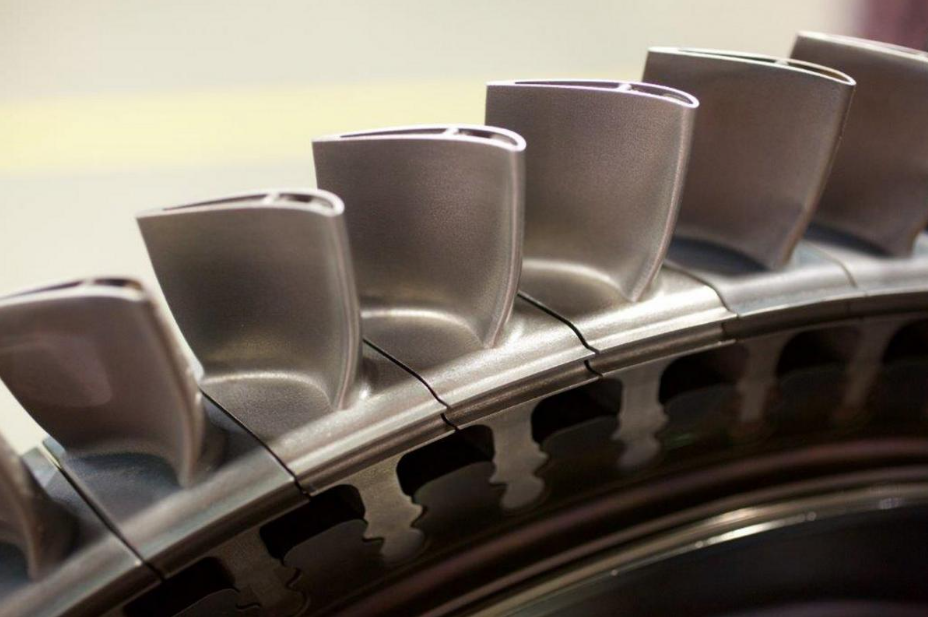
Using a complex shell with many components can make the matching of the heat shield to the outer profile of the shell very complicated . This causes gas to potentially flow upward between components, which is not conducive to cooling the mold shell located in the heating chamber inside the furnace. In turn, repositioning the nozzle downward towards the water-cooled ring can reduce the thermal effect of the inert gas flow on the solidification of the paste region of the casting. The published paper analysis shows that directional solidification methods using cooling gases have high potential. However, there is currently no information on the application of this method to complex ceramic mold production blades with multiple components. Therefore, Sikovok attempted to develop an industrial-scale directional solidification technology for nickel-based superalloy turbine blades using inert Gas Cooling mold shells, called Developed Gas Cooling Casting(DGCC) advanced gas cooling casting method. In this study, the mold shell was cooled by injecting inert gas at supersonic speeds from multiple nozzles located below the heat shield. The use of variable Angle nozzles can correctly direct the flow of inert gas to the surface of a complex shape shell with multiple castings. The study found that the use of gas cooling helped to increase the cooling rate and reduce the primary dendrite arm spacing (PDAS) on the single crystal blade platform compared to conventional radiative cooling in the Bridgman method. The preliminary results show that DGCC gas cooling casting method can be used in industrial scale production to produce high quality single crystal superalloy blades for aero engines.
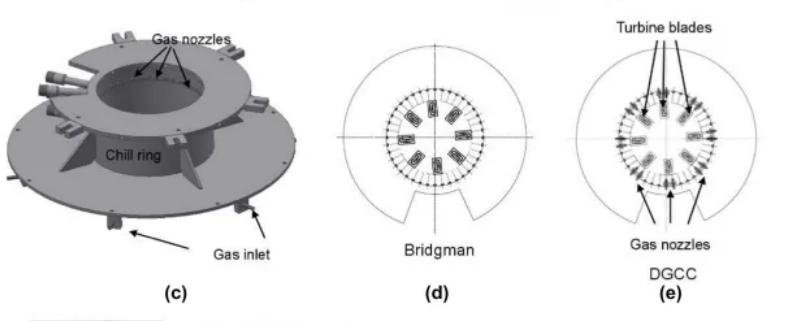
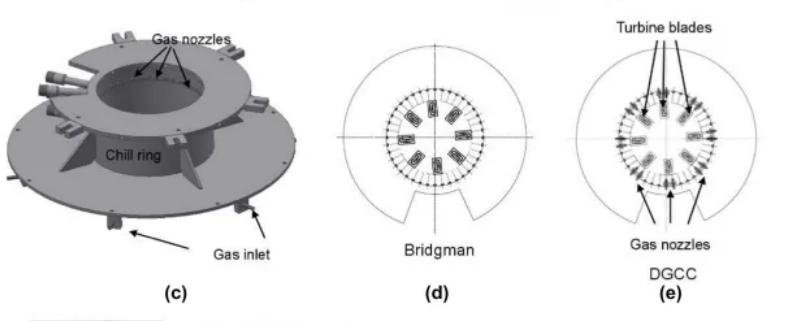
The test castings of CMSX-4 nickel-based superalloys were directionally-solidified using standard Bridgman and DGCC gas cooling casting to produce simulated blades. For this purpose, two kinds of wax mold components were made as the basis for making ceramic mold shells [Figure 1(f) and (g)]. The wax mold assemblies include a 250 mm diameter cooling plate model, a pouring system, a pouring cup, eight simulated blades, and crystal pickers and lifters.
The blades are placed as shown in Figure 1(f). The components are then immersed in a ceramic slurry, followed by alumina particles sprinkled in a fluidized bed to form the first coating of the mold shell. Mullite was used in the second layer. The above two steps were repeated to obtain a total of nine coats, with an average thickness of about 7 mm for the shell wall [Figure 1(g)].
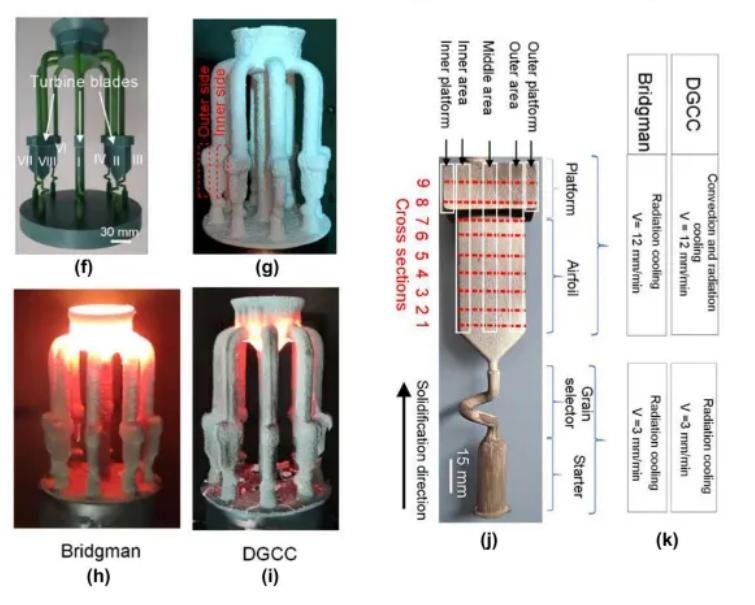
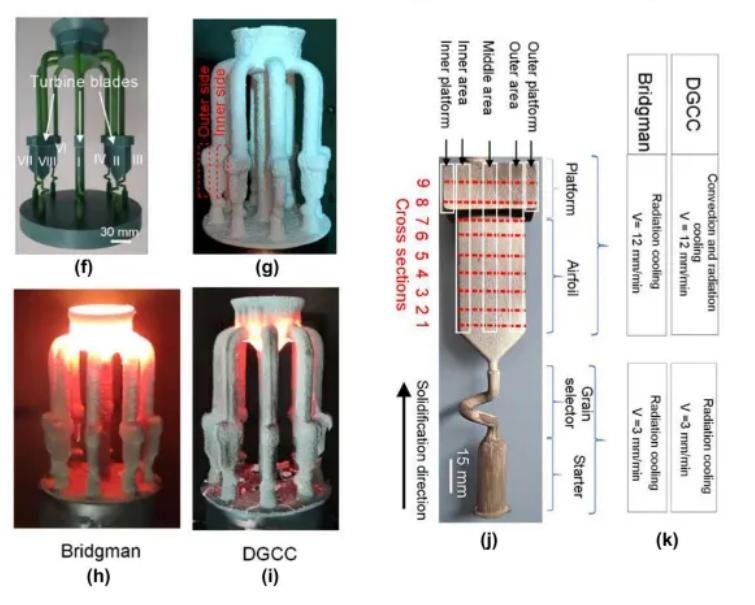
The wax mold melts from the inside of the mold shell, which is then preheated to 800 degrees Celsius. Install the prepared mold shell on the cold plate of the cooling chamber in the furnace [Figure 1(b)]. The first step of directional solidification of single crystal blade was carried out by DGCC gas cooling casting method in JetCaster vacuum induction melting furnace, and argon gas was added to strengthen mold cooling. The furnace consists of a heating and cooling chamber, a mold shell pulling system with a specific speed, and is equipped with a system that can flow inert gases into the cooling chamber [Figure 1(a) to (c)]. The shell is installed on the cooling plate and moved to the heating chamber inside the furnace, which is preheated to 1520 degrees Celsius using a double zone induction heater with a power of 125kw. The heated mold is then filled with CMSX-4 molten nickel-based superalloy of the same temperature and withdrawn at different rates from the heating zone of the furnace to the cooling zone. The pull-out speed is 3 mm/min in the starter and selector regions, and 12 mm/min in the blade region [Figure 1(k)]. In the continuous zone (the transition zone from the separator to the blade), the withdrawal speed gradually increases.
Thank you for your interest in our company! As a professional gas turbine parts manufacturing company, we will continue to be committed to technological innovation and service improvement, to provide more high-quality solutions for customers around the world.If you have any questions, suggestions or cooperation intentions, we are more than happy to help you. Please contact us in the following ways:
- WhatsAPP:+86 135 4409 5201
- E-mail:peter@turbineblade.net