Anti-CMAS Corrosion of Thermal Barrier Coatings for Gas Turbines
Affected by the external environment, some environmental deposits are often produced on the surface of the hot end components of heavy-duty gas turbines (such as turbine blades), mainly from molten salts in the marine environment, vanadates and sulfates, the combustion products of low-grade fuels, and sand, fly ash, runway debris in the air, etc. These deposits have a great impact on the life of the coating. They will penetrate into the coating at high temperatures, causing molten salt corrosion. Therefore, molten salt corrosion is difficult to avoid in the practical application of thermal barrier coatings for heavy-duty gas turbines.
In gas turbines, thermal corrosion is an important form of failure. It is mainly caused by the oxidation of impurities S and some alkali metals in the fuel under high-temperature combustion, and reacting with NaCl, KCl, etc. in the environment to form some molten salt mixtures attached to the surface of the blades, thereby corroding the blades and affecting the life of the blades. Therefore, it is extremely important to improve the thermal corrosion resistance of the coating. Chen et al. [65] studied the hot corrosion resistance of Sc2O3-doped YSZ in Na2SO4/V2O5 (mass fraction 50%∶50%) and found that ScYSZ with a higher Sc2O3 content has better hot corrosion resistance. Yang Yingfei et al. [66] prepared four typical bonding layers, including NiAl coating, NiCoCrAlY coating, Pt-modified NiAl coating and Pt+Hf co-modified NiAl coating, and then coated them with Na2SO4/NaCl (mass fraction 75%∶25%) and conducted hot corrosion tests at 900℃. The results showed that the Pt-modified NiAl coating had the best hot corrosion resistance. CAI et al. [67] used plasma pulse electron beam (HCPEB) to irradiate the CoCrAlY bonding layer and coated it with Na2SO4/K2SO4 (mass fraction 75%:25%) and conducted a hot corrosion test at 900℃. The results showed that irradiation promoted the formation of Al2O3 film in the coating, thereby improving the hot corrosion resistance of the CoCrAlY coating.
CMAS corrosion [68-70] is caused by impurity particles such as sand, dust, and volcanic ash ingested from the air during the use of gas turbines. These impurity particles are mainly composed of CaO, MgO, Al2O3, SiO2, etc., so they are called CMAS. In addition to CaO, MgO, Al2O3, SiO2, etc., CMAS usually contains a small amount of other components, such as Fe2O3 and MgO, so the composition of CMAS is relatively complex. In addition, studies have found that the composition of CMAS will change due to external factors such as location, environment, and climate, causing its melting point to change accordingly, usually within a range of 1,150 to 1,250°C [70-72].
CMAS Corrosion Mechanism
CMAS is very harmful to thermal barrier coatings and seriously affects the life of the coatings, as shown in Figure 7. At low temperatures, the deposited CMAS adheres to the thermal barrier coating of the turbine blades and continuously impacts the coating, causing the coating to wear or even fail [72-73]; at high temperatures, CMAS melts and has two main effects on the YSZ thermal barrier coating, namely, thermochemical and thermomechanical effects.

Thermomechanical aspects[74-75]: After CMAS melts, it penetrates into the ceramic layer under capillary action and solidifies after cooling, filling the pores and cracks of the coating, making the coating denser. The densification of the coating causes the following four damages: first, it reduces the stress tolerance of the coating, increases the Young’s modulus of the coating[76], and accelerates the peeling failure of the coating; second, it reduces the porosity of the coating and increases the thermal conductivity of the coating[77], resulting in a decrease in thermal insulation performance; third, there is a large difference in the thermal expansion coefficient between the CMAS-penetrated area and the non-penetrated area, which promotes thermal stress in the coating during the cooling process and accelerates the peeling of the coating; fourth, it increases the hardness of the coating[78] and reduces the insulation performance of the coating.
Thermochemical aspects[69,79]: At high temperature, CMAS penetrates into the ceramic layer and corrodes the coating mainly through dissolution-reprecipitation, that is, CMAS reacts with the ceramic layer, causing YSZ to dissolve in CMAS and then reprecipitate to form new crystals. On the one hand, the Y-O and Zr-O bonds in the YSZ grains are destroyed during the dissolution process, resulting in an increase in the diffusion rate of Y and Zr. Since the diffusion coefficient of Y ions is larger than that of Zr[80-81], more Y is separated from the YSZ grains, resulting in a lower Y content in some grains. On the other hand, since the solubility of Y2O3 in molten CMAS is higher than that of ZrO2[82], that is, CMAS consumes more Y2O3 in the reaction with YSZ, the reprecipitated crystals have a high ZrO2 content and very little Y2O3. Therefore, these YSZ crystals with less Y content have reduced phase stability due to the lack of stabilizers, and transform from t phase to m phase, causing a 3% to 5% volume change in the coating, thereby changing the stress distribution in the coating and causing the coating to fail. In addition, the reaction degree in different regions is different, and the generated products are different, resulting in different thermal physical properties such as thermal expansion coefficient and elastic modulus, aggravating thermomechanical failure, and thus causing the coating to fail prematurely.
CMAS Corrosion Protection
In recent years, with the development of global industry, the gas inlet temperature of heavy-duty gas turbines has continued to increase, exceeding the melting point of CMAS, causing the CMAS corrosion problem in gas turbines to become more serious, seriously threatening the life of thermal barrier coatings, and attracting more and more attention from researchers around the world. Therefore, it is of great significance to study the CMAS corrosion problem and strive to improve the CMAS corrosion resistance of thermal barrier coatings. In order to enhance the CMAS corrosion resistance of thermal barrier coatings, researchers have mainly conducted a lot of research on ceramic layers in five directions, namely, developing new ceramic layer materials, doping and modifying ceramic layer materials, preparing protective layers on the surface of ceramic layers, designing double-layer ceramic layer structures, and optimizing the surface of ceramic layers.
New ceramic layer materials
The advantages of rare earth zirconate (RE2Zr2O7) [83-84] are high melting point, low thermal conductivity and good phase stability. It is a good choice as a candidate material for thermal barrier coatings and has good resistance to CMAS corrosion. KRÄMER et al. [85] used EB-PVD to prepare a layer of Gd2Zr2O7 (GZO) on an Al2O3 ceramic substrate and conducted a CMAS corrosion test at 1300°C for 4 h. First, it was proposed that the Gd2Zr2O7 ceramic layer has good resistance to CMAS corrosion, and it was proposed that the dissolution of GZO into CMAS is more important than the effect on melt viscosity to promote melt crystallization, that is, GZO inhibits penetration by promoting CMAS crystallization. As shown in Figure 8, it can be clearly seen that compared with the YSZ coating, the Gd2Zr2O7 coating is less damaged under CMAS corrosion. Wang et al. [86] evenly sprinkled Sm2Zr2O7 powder on the surface of CMAS glass (mass ratio of 1:10) and heat treated it at 1350℃ to study the product of Sm2Zr2O7 and CMAS. The results showed that although Sm2Zr2O7 has good resistance to CMAS, when CMAS is excessive, the crystalline product Sm-apatite phase (Ca2Sm8(SiO4)6O2) formed by the reaction of Sm2Zr2O7 and CMAS will continue to decrease until it disappears, destroying the dense structure of the reaction layer, and thus failing to achieve the effect of hindering CMAS penetration.
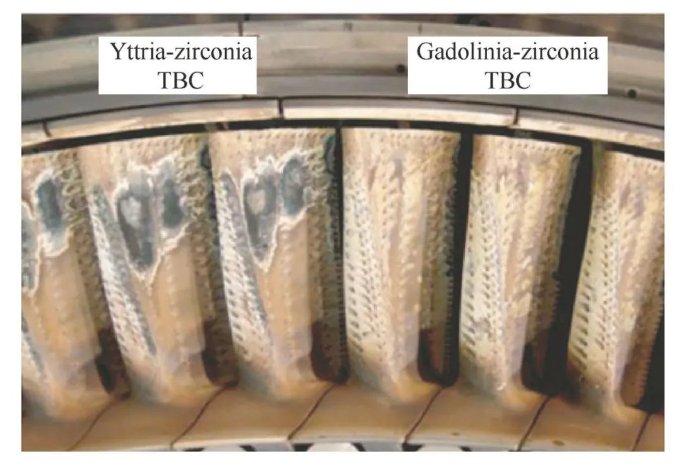
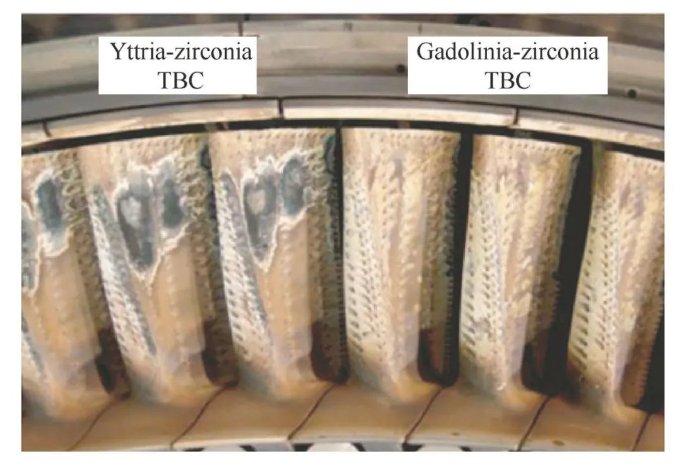
Rare earth phosphate (REPO4) is also a ceramic material with good resistance to CMAS corrosion. GUO et al. [88] used APS to prepare an independent GdPO4 coating and heat treated it at 1250℃ to observe the changes in the organization and microstructure of the coating. The results showed that a dense reaction layer composed of apatite phase, calcium feldspar (CaAl2Si2O8) and spinel phase (MgAl2O4) was formed in the corrosion-corroded coating, which could hinder the continued penetration of CMAS.
In addition, there are also ceramic materials with good resistance to CMAS corrosion, such as La2Ce2O7 [89], RE2Si2O7 [90], and Ti2AlC [91]. TAN et al. [92] prepared Hf6Ta2O17 ceramic layer material by solid phase sintering method, and compared the CMAS corrosion behavior of Hf6Ta2O17 and 8YSZ thermal barrier coatings. The research results show that Hf6Ta2O17 is more resistant to CMAS corrosion than 8YSZ. When CMAS begins to penetrate, a reaction layer and a dense layer will form on the surface of Hf6Ta2O17, which can prevent the molten CMAS from further penetrating.
Doping and modification of thermal barrier coating materials
As is known to all, new ceramic materials such as rare earth zirconates and rare earth phosphates have poor fracture toughness and therefore insufficient thermal shock resistance. Rare earth phosphates are usually doped into rare earth zirconates to improve the fracture toughness of the material and enhance its comprehensive performance. LI et al. [93] used APS technology to prepare a thermal barrier coating (Gd2Zr2O7-LaPO4) doped with 30 wt.% LaPO4 and Gd2Zr2O7, and embedded nano-regions in the microstructure of the coating. The samples were then subjected to CMAS corrosion tests at 1 250°C to study the CMAS corrosion resistance of the coating. The results show that the Gd2Zr2O7-LaPO4 thermal barrier coating has good CMAS corrosion resistance due to its nanostructure, because the reaction between the Gd2Zr2O7-LaPO4 thermal barrier coating and CMAS will form a dense reaction layer to hinder the continued penetration of CMAS, and the nano-regions can gather molten CMAS to enhance the CMAS corrosion resistance of the coating, as shown in Figure 9. LYU et al. [94] used APS to prepare La2Zr2O7 doped YSZ coatings and conducted corrosion tests at 1250℃. The results showed that compared with YSZ coatings and LZ coatings, the LZ doped YSZ thermal barrier coating had the best resistance to CMAS corrosion. FAN et al. [95] used supersonic atmospheric plasma spraying to prepare Sc2O3 doped YSZ (ScYSZ) thermal barrier coatings and then conducted CMAS corrosion tests at 1320℃. The results showed that compared with YSZ coatings, ScYSZ had better phase stability and better resistance to CMAS corrosion. In order to further improve the CMAS corrosion resistance of the coating, it can be achieved by improving the compactness of the ScYSZ coating. In addition, a large number of studies have shown that Al2O3 and TiO2 doped modified YSZ coatings [96-98] also have good resistance to CMAS corrosion.
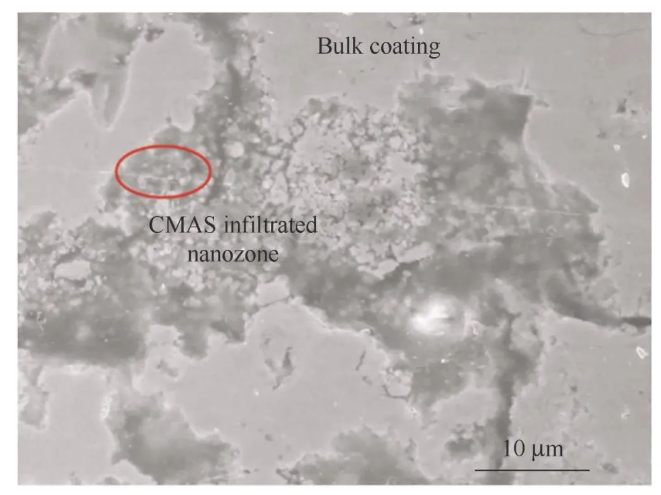
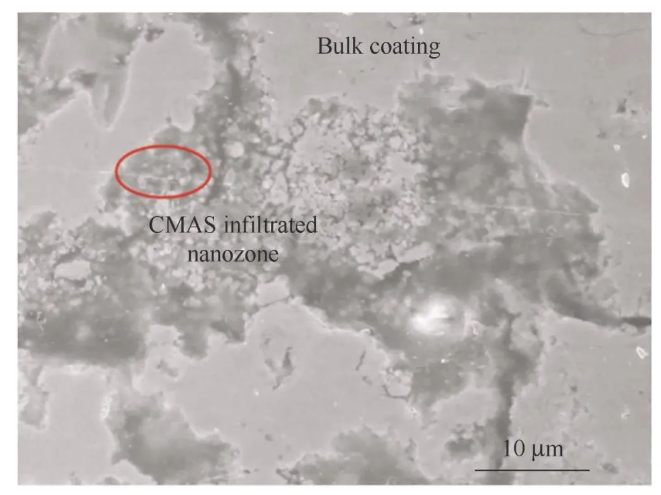