4 Defect formation in DS process
As the structure of advanced turbine blades becomes more complex and larger in size, a variety of solidification defects such as stray grains, freckles, low-angle grain boundaries, streaky crystals, orientation deviation, recrystallization, high-angle grain boundaries, and crystal selection failure are more likely to occur during the single crystal growth process. Casting turbine blades in the form of single crystals is a considerable challenge for foundries.
The problems existing in single crystal turbine blades are mainly concentrated in the growth process, which is closely related to the structure and growth process of single crystal turbine blades. First, the turbine blade body is thin, the tenon is thick and large, the cross-sectional shape is variable, the curvature varies greatly, the internal cooling structure is extremely complex, and there are many microstructures such as air mold holes and spoiler columns, which lead to tortuous and variable dendrite growth paths and drastic changes in growth rate, which can easily cause dendrite torsion and orientation deviation, and induce solidification defects. More seriously, the increase in turbine blade size will extend the growth path of the single crystal, especially at the far end of the water cooling plate. In the later stage of single crystal growth, the temperature gradient decreases sharply with the increase of distance, causing dendrites to grow divergently and increasing the tendency of solidification defects to form [6].
Stray grains
Trapped crystals refer to amorphous regions between grain boundaries or crystals formed by two or more crystals interlacing, colliding or growing together in a material. In the edge plate of the SX turbine blade, the cross section of the casting will experience a sudden change in geometric size, and the temperature field distribution in this area is very complex; during the solidification process of the blade, the alloy undercooling at the edge of the casting exceeds the critical nucleation undercooling of the alloy, resulting in heterogeneous nucleation of impurities at the edge of the casting, forming edge tramp crystals[9].
Previous studies have shown that when the size of the edge plate is small, the higher-order dendrites of the original grains grow into the edge plate, and no tramp crystals are formed. As the size of the edge plate increases, a large number of fine tramp crystals are first formed at the inner corners of the edge plate, and a few tramp crystals grow into the edge plate in the form of dendrites, suppressing the original grains in the center of the edge plate[6]. As the size of the edge plate continues to increase, there is a large undercooling at the edge of the edge plate, the alloy liquid solidifies quickly, and a large solidification shrinkage stress is generated; the heat dissipation performance of the blade-edge plate transition area is poor, the undercooling is small, and the generated dendrites are broken by the shrinkage stress, forming tramp crystals that grow toward the center of the edge plate[9]. According to experimental research, the reduction of platform height, the increase of platform length, the outer side of the platform, and the alloy composition with high content of refractory elements (Re, W, Ta, Hf) all increase the tendency of the formation of impurity crystals[10].
The formation of impurity crystals on the edge plate can be controlled by optimizing the directional solidification process (reducing the solidification rate), local cladding treatment (coating with thermal resistance materials), and adding a seeding system.
Freckle
In the later stage of the growth of single crystal blades, especially at a distance from the water cooling plate, it is easy to form some chain-like, fine equiaxed grains parallel to the crystal growth direction. Because the defect surface after macroscopic corrosion shows obvious spots, it is called freckles or freckle chains. At present, the structure of turbine blades tends to be complicated, and the content of high-melting-point alloy elements in the alloy continues to increase, which leads to an increase in the tendency of freckle formation.
The formation mechanism of freckles is mainly caused by the convection of alloy liquid caused by solute segregation during solidification, and is also related to the remelting of secondary dendrites and the deflection of primary dendrites. In the DS process, W and Re are enriched in the dendrite stem area, and Al and Ta are enriched in the alloy liquid between dendrites. There is a density difference between the former and the latter. As the mushy zone solidifies, the difference between the density of the alloy liquid in the mushy zone and the density of the liquid at the solidification front increases. The density distribution of heavy top and light bottom causes the alloy liquid in the mushy zone to be subject to upward buoyancy. When the viscous resistance of the alloy liquid in the mushy zone is exceeded, the alloy liquid in the mushy zone will convect between the dendrites and form a convection channel of a certain width in the mushy zone. The flow of this alloy liquid will melt or break the dendrites to form dendrite fragments. If these dendrite fragments do not have time to flow out of the channel with the alloy liquid and remain in the channel, they will form spots on the casting surface as the channel solidifies[11].
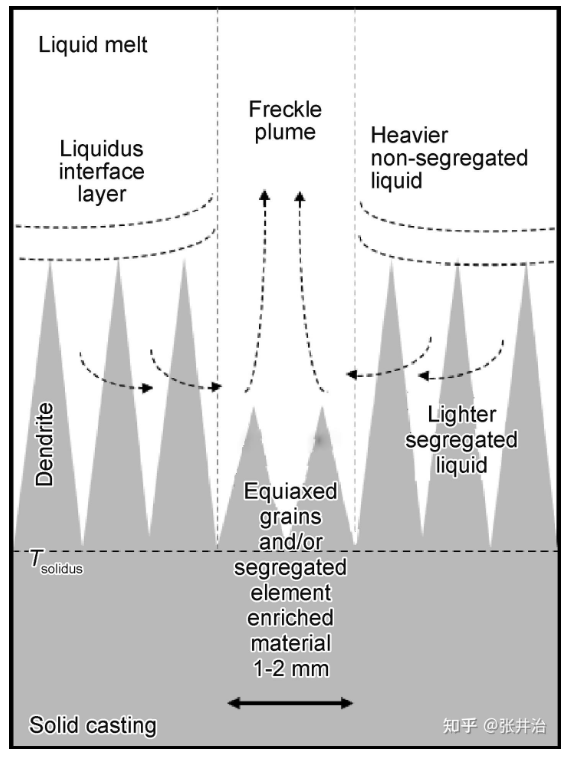
Increasing the content of Ta and Al alloy components and reducing the content of W and Re can help reduce the tendency of freckle formation. In the DS process, increasing the pulling rate and increasing the temperature gradient can reduce the tendency of freckle formation. Vibration can significantly weaken the convection of the liquid phase during directional solidification, thereby reducing the tendency of freckle formation.
Low Angle Grain Boundary
The formation of low-angle grain boundaries is related to the orientation deviation of dendrites caused by dendrite deformation: (1) Thermomechanical stress generated by the precipitation of γ’ phase during steady-state growth; (2) The obstruction and extrusion of the mold shell cause shrinkage stress in the dendrites; (3) The solute convection caused by the uneven temperature field in the mushy zone and the asymmetric force on the dendrites lead to plastic deformation of the dendrites, which triggers the cumulative change of dendrite orientation. The low-angle grain boundary is formed at the junction of the deflected dendrite and the original non-deflected dendrite.
When a large-sized single crystal blade solidifies, it is difficult for the S/L interface to maintain a planar state (concave when the pulling speed is high and convex when the pulling speed is low). The temperature gradient direction of the non-straight S/L interface does not coincide with the axial direction of the specimen. Any fluctuations in the solidification process may cause orientation changes, thereby forming low-angle grain boundaries. These fluctuations may cause some dendrites to grow in a non-steady state during the growth process from the expansion zone to the blade body, resulting in the angle of the low-angle grain boundary of the blade body mainly concentrated in the range of 2°-6°. This is determined by the solidification properties of the alloy, and it is difficult to find a reasonable way to avoid it [12].
The number of small-angle grain boundaries in the extension zone is significantly lower than that in the blade body, and the misorientation angle is also much smaller, but the number of locations with misorientation angles less than 2° in the extension zone and the blade body is comparable, indicating that they have comparable abilities to produce small misorientations. This is because the extension zone is in the early stages of single crystal growth, and most dendrites show steady-state growth, while the number of dendrites in steady-state growth in the extension zone and the blade body is comparable.
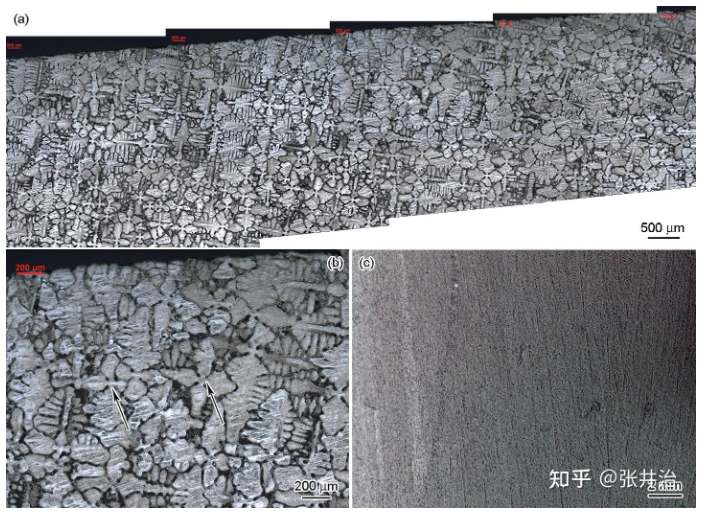
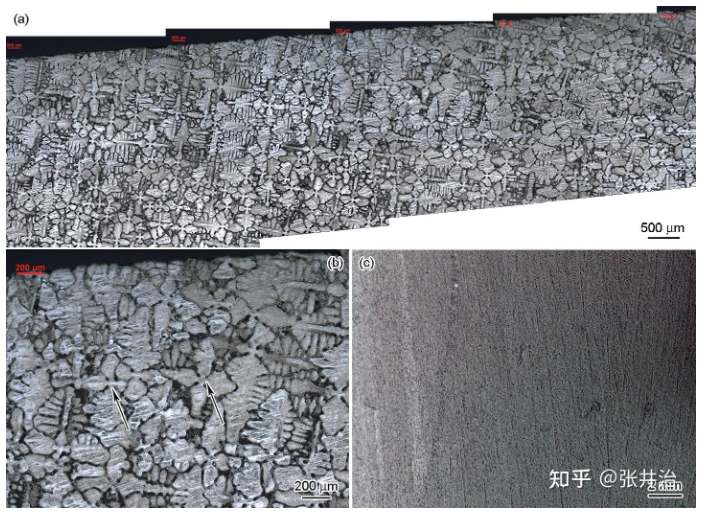
Striped crystal
Striated crystals are a type of narrow linear defect on the surface of a casting, mostly occurring on the upper part of the blade of a casting. They are generally about 1 mm wide and several to tens of mm long, with an identifiable starting position. They generally disappear after growing for a few centimeters, but they can also expand laterally to the entire blade, developing from a linear defect to a three-dimensional large-scale defect and transforming into a miscellaneous crystal defect. The direction of the streak crystal is always basically consistent with the growth direction of the dendrite at that location.
The appearance of streak crystals is due to the fact that the main trunk of a single dendrite on the surface of the casting is torn off in the mushy zone, but is welded by the residual liquid, showing an obvious starting point. The main reason for this tearing is that the dendrite shrinkage caused by the adhesion of the shell is severely hindered or the dendrite strength is severely damaged due to the cutting of the inclusion. The torn dendrite will undergo a certain degree of overall deflection, forming a narrow grain enclosed by a small-angle grain boundary on the matrix structure [12].
Recrystallization
SX is mainly composed of γ phase and γ’ phase in the form of eutectic combination. When the local energy is high due to the concentration of deformation stress in the local area of the surface, and then when it reaches a certain temperature in the subsequent heating, the γ’ phase dissolves in the single crystal alloy. After dissolution, it is very easy to form a cellular structure in the γ phase dissolution area. The surface recrystallization of SX first starts in the dendrite rod area of the surface. The initial organization is cellular. Then the grains begin to grow gradually into the eutectic phase γ/γ’ area containing coarse γ’ phase. The growth of recrystallized grains is accompanied by a clear interface between the γ’ crystal grains and the matrix [13]. An important condition for the formation of recrystallized grains: the dissolution of the cast γ’ phase.