Hot Corrosion Mechanism of Turbine Disk High Temperature Alloy
The high-temperature alloys used for aircraft engine turbine disks are deformable high-temperature alloys (such as Inconel 718, GH4169, etc.) and powder high-temperature alloys (such as RR1000, ME3, FGH96, etc.). The service temperature of deformed high-temperature alloy turbine discs is usually not higher than 650 ℃, while the service temperature of powder high-temperature alloy turbine discs is usually not higher than 750 ℃. The thermal corrosion that occurs under the coupling of marine environment and working conditions is mainly low-temperature thermal corrosion. At present, domestic and foreign scholars mainly study the hot corrosion mechanism of turbine disk alloys by hot corrosion of turbine disk alloys in Na2SO4+NaCl or Na2SO4+NaCl+V2O5 with a certain mass fraction ratio, combined with characterization of the microstructure and composition of the hot corrosion layer. Mahobia et al. [42,43,44] studied Inconel 718 and GH4169 alloys and found that during the hot corrosion process of Inconel 718 and GH4169 alloys, chloride ions in NaCl can damage the oxide layer and penetrate into the matrix, forming metal chloride MCl, which then evaporates from the surface to form pits, providing channels for the flow of corrosive media. Subsequently, chloride ions can induce internal corrosion, causing self-sustaining chlorination and oxidation of metal elements, while the invasion of sulfur elements can also cause internal sulfurization of the alloy, exacerbating hot corrosion. As shown in Figure 5 (a), with the invasion of S element, the needle shaped δ phase (Ni3Nb) in GH4169 will undergo sulfurization and accumulate inside the corrosion layer. In addition, the low melting point sulfide Ni3S2 (melting point 787 ℃) generated by the reaction can form a lower melting point eutectic with metal Ni (melting point 645 ℃), and the liquid sulfide eutectic can infiltrate into the metal along the grain boundary; In addition, the number of crystal defects in liquid sulfides and their co crystals with Ni is much higher than that in oxides, providing a fast channel for metal diffusion and leading to rapid damage of the matrix alloy. The hot corrosion of IN718 high-temperature alloy in Na2SO4+NaCl+V2O5 is mainly caused by oxidation process and vanadium corrosion caused by V2O5. The hot corrosion mechanism model is shown in Figure 5 (b). In Long Xiaoqing’s research [45], it was discussed that liquid V2O5 has a strong thermal corrosion effect in the working environment of gas turbine engines. V2O5 mainly comes from the alloy material itself or fuel, and the vanadium corrosion it produces will damage the continuity and integrity of the Cr2O3 (or Al2O3) oxide film, causing the oxide film to lose its protective effect [46]. After hot corrosion in Na2SO4+NaCl+V2O5 medium, the surface of the alloy undergoes sulfurization and acid-base melting with Na2SO4, and the substrate alloy under the original oxide layer forms a lean aluminum or chromium region. A protective oxide film is no longer formed to repair the damaged protective layer. The outer oxide film that loses its protective properties becomes more porous and easily peeled off due to the infiltration of Na2SO4, resulting in accelerated oxidation. The research by Pradhan [47], Mahobia et al. [42] shows that the thermal corrosion of Inconel 718 alloy is more severe in Na2SO4+NaCl+V2O5 corrosive medium than in Na2SO4+NaCl. The formation of different vanadate compounds occurs together with the formation of Na2Cr2O7, where sulfur ions react with chromium, iron, nickel, or their oxides to form sulfides.
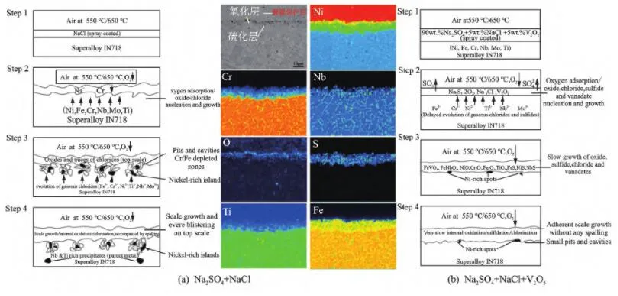
For powder high-temperature alloys, their low-temperature hot corrosion process is similar to that of deformed high-temperature alloys. In addition to characterizing the oxidation and sulfurization products in the hot corrosion layer of powder high-temperature alloys, domestic and foreign scholars also characterize the damage evolution of the hot corrosion process from the depth of oxidation, surface corrosion morphology, and corrosion pit depth/diameter. As shown in Figure 6 (a), Encinas Oropesa et al. [48] measured the oxide thickness and determined the oxidation damage characteristics of RR1000 alloy using thermogravimetric analyzer, focused ion beam (FIB), and scanning electron microscope. The results showed that the thickness of chromium/titanium oxide formed by surface oxidation of RR1000 alloy increased parabolically with time, and the degree of hot corrosion damage increased with the increase of salt deposition rate, but decreased with the increase of Cl content in salt deposition rate. Birbilis et al. [49] quantitatively analyzed the evolution of characteristic dimensions such as depth and equivalent diameter of corrosion pits on the surface of Rene 104 alloy using optical contour measurement method, and proposed that an interpolation empirical model can be further established to study the growth kinetics of corrosion damage. As shown in Figure 6 (b), based on the quantitative analysis of corrosion pit depth, it can be seen that low-temperature hot corrosion has a latent period (about 5.5 hours) before large-scale damage accumulation, and the evolution of corrosion damage is manifested as pitting corrosion caused by selective carbide oxidation. As hot corrosion continues, the damage on the alloy surface is determined by sulfur related corrosion due to the lack of chromium oxide. In this oxidation and sulfurization hot corrosion process, Gabb et al. from NASA Glenn Research Center [50] also found that the grain boundaries inside the corrosion pits are often preferentially eroded.
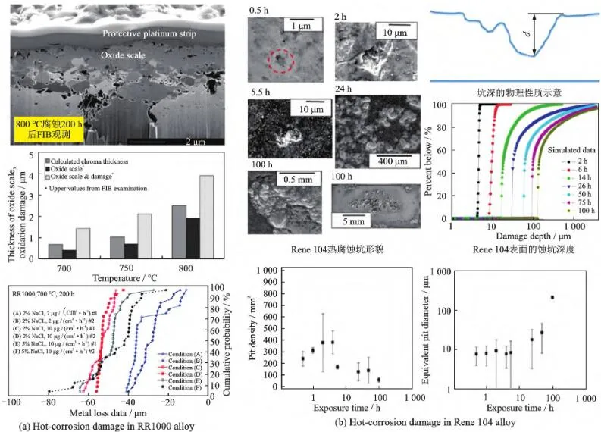
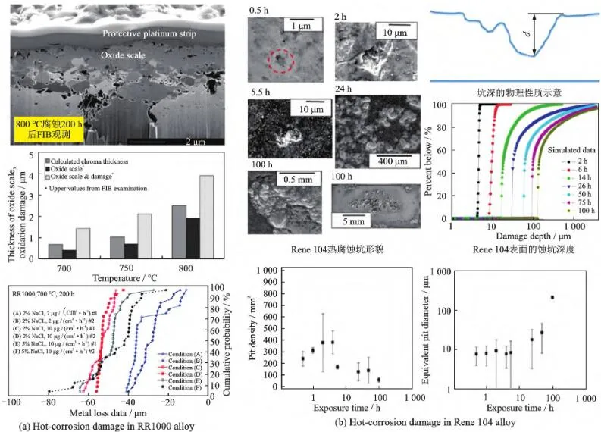